Energy in Transition
Rice is leveraging its core research strengths in engineering and science — and its location in the U.S. energy capital — to tackle the urgent challenges of global climate change.
By David Levin, Mike Williams, Avery Ruxer Franklin and Lynn Gosnell
Illustration by Dan Page
What do we mean when we talk about energy transition? At Rice, the term signifies a multifaceted approach to research that aims to decarbonize our energy systems. Because of Rice’s location in Houston, the fossil fuel energy capital of the U.S., our scholars are uniquely situated to address the urgent need for both immediate and long-term climate solutions.
When Marcia O’Malley, a mechanical engineer and the associate dean for research and innovation at Rice, thinks of the complex challenges inherent in making energy more sustainable, she imagines a flow chart filled with questions and capabilities. What long-timescale solutions can help us reduce our reliance on fossil fuel-based energy sources? Where are we getting our sources of energy, and how does location differentially impact communities and neighborhoods? What new technologies will improve energy production? How do we produce, store and transport alternative energies like solar, wind and geothermal? How do we model, predict and adapt to the impacts of climate change, both locally and globally? What technologies will help us consume less energy? How do we capture and sequester carbon?
Universities like Rice are perfectly situated to address these complex problems, O’Malley says. “Where else do you have so many different perspectives and research strengths?” In the following roundup, we take a look at six current Rice research projects that offer intriguing answers from the engineering and science perspective to some of these challenges.
Safer CO2 Storage With Fiber Optics
Researchers are studying ways to improve underground carbon dioxide storage.
These days, carbon capture has become something of a buzzword. The technology seeks to remove greenhouse gases like carbon dioxide from smokestacks and vehicles before it can reach the atmosphere, where it contributes heavily to climate change. In theory, it’s a sound idea — but what do you do with all that CO2 once you extract it?
One option: Store it underground. The concept is already being tried under the bottom of the North Sea and in various sites around the continental U.S. It involves injecting CO2 thousands of feet down into bedrock, where it will be absorbed by existing reservoirs of salty water. The challenge, however, is that seismic activity could break the reservoirs open, release the gas from cracks and fault lines, and contaminate nearby groundwater.
Geoscientist Jonathan Ajo-Franklin wants to prevent these catastrophes. He’s testing a new way to monitor huge areas of land for even the most miniscule seismic activity and is using a novel method: sensors made from miles of fiber-optic cable.
Traditional methods use seismometers — a type of microphone that listens for rumbles in the Earth — to detect small earthquakes as they occur. Each of those sensors only represents a single point on the ground, however. With fiber-optic sensors, Ajo-Franklin’s team can measure movement constantly along a line stretching 25 miles.
The devices work thanks to a quirk of the cable itself. Shine a laser in one end, and you’ll see a pulse of light moving through it. If you shake the cable at any point, however, the light will scatter, sending a small amount back up the way it came. By measuring how much of this scattering occurs — and how long it takes for that light to return to its source — Ajo-Franklin can record not only the intensity of a seismic event, but also exactly where it occurred along the cable with incredible precision.
This sort of technology could be a boon for monitoring CO2 reservoirs, he adds. By lowering a single half-inch-wide cable into a bore hole, it may be possible to detect tiny movements along the entire depth of the containment site.
In addition to testing and monitoring CO2 reservoirs, he says, the technology could also be used to identify sites for hydrothermal energy — which involves circulating water through volcanically heated rock — or could be installed onto existing communication networks above ground, effectively turning them into massive seismic detectors that span entire counties.
“You can get a massive amount of seismic data from a fiber the size of a human hair,” he says. “It’s pretty remarkable.” — David Levin
Jonathan Ajo-Franklin is a professor in the Department of Earth, Environmental and Planetary Sciences.
A New Way to Harness the Sun’s Energy
A crystal structure called perovskite may hold the key to better solar collectors.
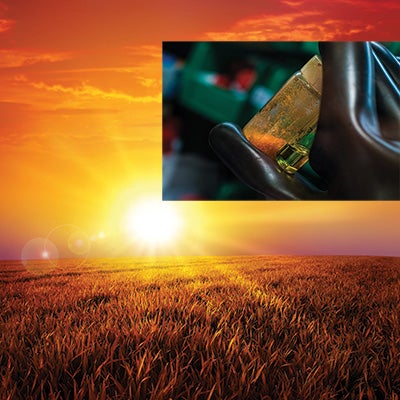
Since their commercial introduction in the 1950s, solar photovoltaic panels have been a promising source of clean energy. While these panels, which are largely made of silicon, have improved dramatically over the past few decades, they still can only eke out between 18% and 22% efficiency, at best.
A crystal structure called perovskite could change this, however. When used to create solar panels, perovskite crystals — which can be made of many different chemical substances — can generate up to 30% efficiency, says chemical engineer Aditya Mohite. “The only challenge is that as a crystal, it’s pretty unstable,” he says. “It can degrade easily with moisture. If you want to put a panel on your roof, it has to last for 20–25 years.”
It’s also a bear to work with: In order to squeeze peak efficiency from a perovskite crystal, it has to be laid down into thin, uniform layers, but it has a natural tendency to clump up as it’s being applied. The result is lower electrical output.
Mohite and his collaborator, polymer physicist Amanda Marciel, may have found a way to tame perovskite. The pair dissolved perovskites made from a chemical called methylammonium lead halide into a chemical solution, then used that solution to regrow a new batch of crystals. Shockingly, the perovskite started to behave, forming flat, uniform sheets with ease.
“The solution seemed to retain the original crystal’s behavior,” says Marciel. “It didn’t break down into its individual components — instead, it formed into tiny crystal ‘seeds’ that have the same properties as the parent crystal.” From those, the pair were able to grow the crystals into a thin, flexible film, she says.
Sandwiching these films between layers of organic molecules, the researchers found, could improve the perovskite’s efficiency and stability, Mohite adds. “This is a huge step toward long-lasting, robust solar cells. And since they’re flexible, they open up all sorts of new applications. You could imagine wrapping an entire structure in panels like these.” — David Levin
Aditya Mohite is an associate professor in the Department of Chemical and Biomolecular Engineering and an associate professor in the Department of Materials Science and Nanoengineering. Amanda Marciel is the William Marsh Rice Trustee Chair and an assistant professor in the Department of Chemical and Biomolecular Engineering.
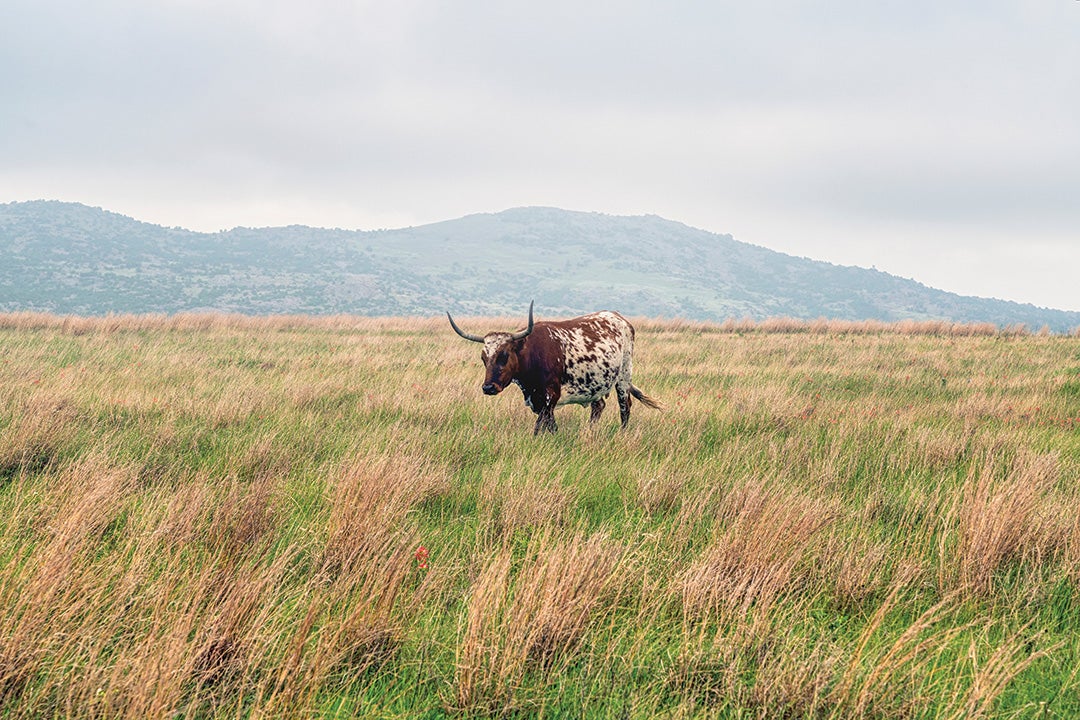
Underground Economy
Rice scholars are developing new standards to make carbon sequestration a valuable property right for landowners.
Across rural Texas, miles and miles of grassland prairies, savannah and shrub offer diverse habitats for wildlife, grazing lands for livestock and recreational opportunities for families. These valuable natural resources support key economic enterprises such as ranching and farming. Environmentalist, Rice professor and attorney Jim Blackburn sees a new kind of enterprise in these iconic landscapes — one that sequesters and monetizes organic carbon in the loam and clay of Texas soils.
Along with colleagues Carrie Masiello, a Rice soil scientist and the W. Maurice Ewing Professor of Biogeochemistry, and Ken Medlock, senior director of the Center for Energy Studies at the Baker Institute for Public Policy, as well as other stakeholders, he has developed a protocol called BCarbon, which measures, verifies and certifies soil carbon capture for carbon credit trading. The newly created market essentially pays landowners to pull carbon dioxide from the atmosphere and store it in the dirt.
“BCarbon is practical, works for landowners and is absolutely needed now in our attempts to address atmospheric buildup of carbon dioxide,” Blackburn says. “This effort has the potential to transform ranching and farming in the U.S., restore ecological systems in one of the largest land conservation and restoration programs in United States history, and help carbon emitters by providing lower-cost carbon capture and storage options. It is a win-win-win concept.”
— Lynn Gosnell
To learn more about soil carbon sequestration and the BCarbon standards, check out our Q&A with Jim Blackburn.
Jim Blackburn is a professor in the practice of environmental law in Rice’s Department of Civil and Environmental Engineering and the co-director of the Severe Storm Prediction, Education and Evacuation from Disasters Center. He is a faculty scholar at Rice’s Baker Institute for Public Policy and a practicing environmental attorney.
Through a Glass, Luminescently
Rice engineers propose a new method for converting sun-light to electricity.
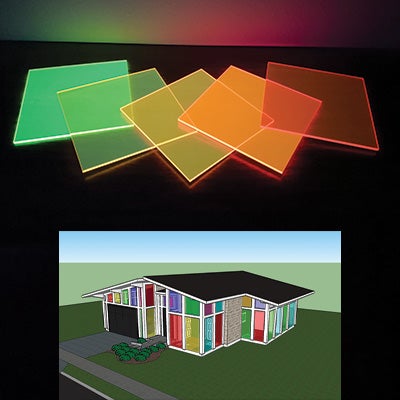
Rice engineers have suggested a colorful solution to next-generation energy collection: luminescent solar concentrators (LSCs) in windows. Led by chemical engineer Rafael Verduzco ’01 and postdoctoral researcher and lead author Yilin Li, the research team designed and built colorful, foot-square “windows” that sandwich a conjugated polymer between two clear acrylic panels. The thin middle layer is designed to absorb light in a specific wavelength and guide it to solar cells that line the edges of the panel.
“The motivation for this research is to solve energy issues for buildings through integrated photovoltaics,” said Li, who began the project as part of a “smart glass” competition. “Right now, solar rooftops are the mainstream solution, but you need to orient them toward the sun to maximize their efficiency, and their appearance isn’t very pleasing. We thought, why can’t we make colorful, transparent or translucent solar collectors and apply them to the outside of buildings?”
While the amount of juice generated by the test units is far less than that collected by even average commercial solar cells, which routinely convert about 20% of sunlight into electricity, LSC windows never stop working. They recycle light from inside the building into electricity when the sun goes down. In fact, tests showed they were more efficient at converting ambient light from LEDs than they were from direct sunlight, even though the sunlight was 100 times stronger.
“Even indoors, if you hold up a panel, you can see very strong photoluminescence on the edge,” Li said. The panels he tested showed a power conversion efficiency of up to 2.9% in direct sunlight and 3.6% under ambient LED light.
Various types of luminophores (atoms in a chemical compound responsible for its luminescent properties) have been developed over the last decade, but rarely with conjugated polymers, according to Verduzco. “We’ve learned a lot about improving the stability of conjugated polymers in recent years, and in the future, we can engineer the polymers for both stability and desired optical properties,” Verduzco said. The study appears in the journal Polymer International. Research was supported by Solera City Energy.
— Mike Williams
Rafael Verduzco is a professor in the Department of Chemical and Biomolecular Engineering and the Department of Materials Science and Nanoengineering.
A Renewable Energy Model
A Rice study shows how proposed projects could eliminate the need for coal and drastically cut pollution.
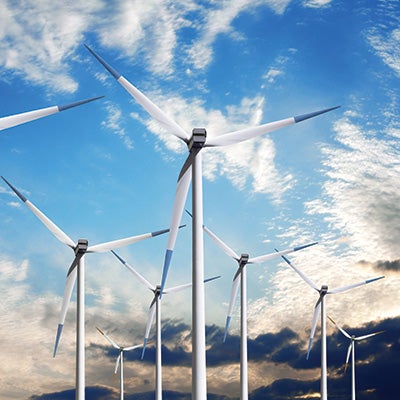
Texas can be a model for the nation on how to effectively replace coal with wind and solar for the state’s energy needs while meeting environmental goals. Environmental engineer Daniel Cohan and computer science major Richard Morse ’22 used optimization modeling to identify the most cost-efficient combinations of proposed wind and solar projects that have the potential to replace coal-fired power generation in Texas.
The team’s research found that just a third of the wind and solar projects already proposed to the Electric Reliability Council of Texas (ERCOT) as of June 2020 could replace almost all of the state’s coal generation. Since June 2020, dozens of those projects have been built, and the queue of proposed solar projects has doubled.
“Transitioning away from coal as quickly as possible is imperative,” said Cohan. “Texas still burns more coal and emits more sulfur dioxide and other pollution than any other state,” he said. Several large Texas coal plants closed in 2018, and most remaining plants have operated far below capacity, an issue that came to the forefront amid the hard freeze and subsequent outages of February 2021. The key to success for wind and solar will be an expansion of transmission lines connecting the windiest and sunniest parts of the state to cities.
The study shows that wind power from West Texas tends to peak overnight, whereas South Texas wind peaks with sea breezes on summer afternoons and evenings. Together with daytime solar, these complementary sources can cover most, but not all, hours of the day throughout the year. Cohan noted that natural gas will continue to be critical to Texas’ electric reliability in the near term, especially as ERCOT works to recover from the 2021 freeze.
“Even with complementary siting, there will still be hours when the sun isn’t shining and the wind isn’t blowing,” Cohan said. “Historically, the main challenge has been summer afternoons, when air conditioners are running full blast, and conversely, the occasional deep freeze. Solar and coastal winds perform well during summer peaks but can have lulls on some evenings when we’ll need something else to kick in.”
“Cleaning up the energy that we use to power society is one of the most important things we need to do,” said Morse, who won the Malcolm Verdict Memorial Poster Competition at the Texas Energy Summit in 2020 for his work on the project.
— Mike Williams
Daniel Cohan is an associate professor of civil and environmental engineering. Richard Morse graduated this May with a degree in computer science and computational and applied mathematics. This research was published in “Renewables: Wind, Water, and Solar.” Co-authors of the paper are Rice undergraduate civil engineering major Sarah Salvatore ’23 and mathematics and philosophy major Joanna Slusarewicz ’20. The Energy Foundation supported the research
Stronger, Tougher, Cleaner
At Rice’s Carbon Hub, researchers are creating new sustainable materials to fight climate change.
Steel-making is one of the most carbon-intensive processes there is. Each year, steel plants release nearly 3 billion tons of carbon dioxide, or about 8% of global CO2 emissions. That puts the steel industry somewhere between India and the United States in terms of greenhouse gas pollution.
At the moment, there aren’t many materials available that share the same traits as steel. Steel is strong, easy to manipulate and relatively cheap, a trifecta that has made it an essential material for global infrastructure.
Chemical engineer Matteo Pasquali thinks bridges and buildings made from steel columns could someday be a relic of the past. He’s directing the Rice Carbon Hub, a research initiative focused on making products out of carbon while co-producing clean hydrogen.
Within the Carbon Hub, his team is working to efficiently turn natural gas into new materials based on carbon nanotube fibers. “These fibers are kind of the best of all worlds — as a material, they’re stronger than steel, tougher than Kevlar and, on a mass basis, more conductive than copper,” he says.
Since they’re made directly from hydrocarbons, the molecules that make up fossil fuels, these materials also come with a distinct advantage over steel: Instead of releasing CO2 into the atmosphere, the manufacturing process never generates CO2 at all, because the carbon makes up the material itself.
“Basically, that means we can lower CO2 emissions and fight climate change by using hydrocarbons to make sustainable materials,” Pasquali says. “If we use fossil hydrocarbons as feedstock, we reduce or eliminate CO2 emissions; if we use biogas, these materials could be a net sink of CO2. And, of course, hydrogen is a very valuable coproduct.”
Scaling up materials manufacturing and attaining industrial efficiency takes years. In the meantime, Pasquali is collaborating with other researchers at the Carbon Hub to find new uses for carbon nanotube fibers, with success. Two years ago, they reported joint research with the Texas Heart Institute and Baylor College of Medicine, where Rice’s carbon nanotube fibers were used to rewire damaged hearts. More recently, electrical engineer Junichiro Kono used carbon nanotube fibers sewn into fabrics to turn heat into electrical energy. Among other uses, this application could eventually lead to wearable medical sensors that don’t require batteries.
The Carbon Hub work goes beyond carbon nanotube materials and includes research on carbon by bioscientists Carrie Masiello, Daniel Cohan and Pedro Alvarez that can be used to improve soil; work by architect Juan José Castellon and civil engineer and Rice President Reginald DesRoches on carbon-based materials for buildings; as well as work by mechanical engineer Geoff Wehmeyer
and physicists Matthew Foster and Douglas Natelson on carbon-based electrical conductors.
— David Levin
Matteo Pasquali is the A.J. Hartsook Professor in the Department of Chemical and Biomolecular Engineering, the Department of Chemistry, and the Department of Materials Science and Nanoengineering, and the director of the Carbon Hub. Junichiro Kono is the Karl F. Hasselmann Chair in Engineering and a professor in the Department of Electrical and Computer Engineering, the Department of Physics and Astronomy, and the Department of Materials Science and Nanoengineering.